Cyclic AMP and the Control of Airways Smooth Muscle Tone
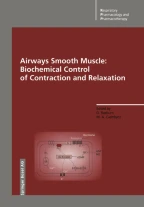
As little as a decade ago, most information concerning the role of cyclic adenosine 3′,5′-monophosphate (cyclic AMP) as a second messenger mediating bronchorelaxation was inferred from studies on vascular smooth muscle. Much has changed since that time. Airways smooth muscle itself has become the target of considerable scientific investigation focused on the molecular mechanisms responsible for the relaxant activity of β-adrenoceptor agonists and other agents that activate adenylyl cyclase. In many ways the information gained from thes studies reinforces the traditional concept that the action of β-adrenoceptor agonists on airways smooth muscle is mediated by a cyclic AMP-regulated protein phosphorylation cascade system, with cyclic AMP-dependent protein kinase (protein kinase A) serving as the intra- cellular target enzyme for cyclic AMP. Recently, however, data have emerged that suggest an alternative mechanism by which cyclic AMP can relax airways smooth muscle. Still other data have prompted th near-heretical proposal that bronchorelaxation in response to β-adrenoceptor agonists is mediated, at least in part, by a cyclic AMP-independent mechanism.
This is a preview of subscription content, log in via an institution to check access.
Access this chapter
Subscribe and save
Springer+ Basic
€32.70 /Month
- Get 10 units per month
- Download Article/Chapter or eBook
- 1 Unit = 1 Article or 1 Chapter
- Cancel anytime
Buy Now
Price includes VAT (France)
eBook EUR 85.59 Price includes VAT (France)
Softcover Book EUR 105.49 Price includes VAT (France)
Tax calculation will be finalised at checkout
Purchases are for personal use only
Preview
Similar content being viewed by others
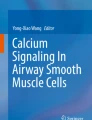
Regulation of Airway Smooth Muscle Contraction by Ca2+ Signaling: Physiology Revealed by Microscopy Studies of Lung Slices
Chapter © 2014
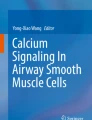
Ca2+ Signaling and P2 Receptors in Airway Smooth Muscle
Chapter © 2014
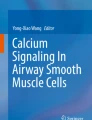
Chapter © 2014
References
- Birnbaumer L, Brown AM. G-Proteins and the mechanism of action of hormones, neurotransmitters and autocrine and paracrine regulatory factors. Am Rev Resp Dis 1990; 141: S106–S114. ArticlePubMedCASGoogle Scholar
- Flockhard DA, Corbin JD. Regulatory mechanisms in the control of protein kinases. CRC Critical Rev Biochem 1982; 12: 133–86. ArticleGoogle Scholar
- Giembycz MA, Raeburn D. Putative substrates for cyclic nucleotide-dependent protein kinase and the control of airway smooth muscle. J Auton Pharmacol 1991; 11: 365–98. ArticlePubMedCASGoogle Scholar
- Torphy TJ. Biochemical regulation of airway smooth muscle tone: current knowledge and therapeutic implications. Rev Clin Basic Pharmacol 1987; 6: 61–103. CASGoogle Scholar
- Beavo JA, Multiple isozymes of cyclic nucleotide phosphodiesterase. Adv Second Messenger Phosphoprotein Res 1988; 22: 1–38. PubMedCASGoogle Scholar
- Torphy TJ, Undem BJ. Phosphodiesterase inhibitors: new opportunities for the treatment of asthma. Thorax 1991; 46: 512–23. ArticlePubMedCASGoogle Scholar
- Krebs EG. The mechanism of hormonal regulation by cyclic AMP. In: Endocrinology. Proceedings of the 4th International Congress. Amsterdam: Excerpta Medica, 1973: 17–29. Google Scholar
- Robison GA, Butcher RW, Sutherland EW. Cyclic AMP and hormone action. In: Cyclic AMP. New York: Academic Press, 1971: 17–47. Google Scholar
- Rinard GA, Jensen A. Preparation of hormone-sensitive airway smooth muscle adenylate cyclase from dissociated canine trachealis cells. Biochim Biophys Acta 1981; 678: 207–12. ArticlePubMedCASGoogle Scholar
- Jones CA, Madison JM, Tom-Moy M, Brown JK. Muscarinic cholinergic inhibitors of adenylyl cyclase in airway smooth muscle. Am J Physiol 1987; 253: C97–C104. PubMedCASGoogle Scholar
- Murad F, Kimura H. Cyclic nucleotide levels in incubations of guinea-pig trachea. Biochim Biophys Acta 1974; 343: 275–86. ArticlePubMedCASGoogle Scholar
- Rinard GA, Rubinfeld AR, Brunton LL, Mayer SE. Depressed cyclic AMP levels in airway smooth muscle from asthmatic dogs. Proc Natl Acad Sci 1979; 76: 1472–6. ArticlePubMedCASGoogle Scholar
- Wong SK, Buckner CK. Studies of β-adrenoceptors mediating changes in mechanical events and adenosine-3′,5′-monophosphate levels in guinea-pig trachea. Eur J Pharmacol 1978; 47: 273–80. ArticlePubMedCASGoogle Scholar
- Zhou H-L, Newsholme SJ, Torphy TJ. Agonist-related differences in the relationship between cAMP content and protein kinase activity in canine trachealis. J Pharmacol Exp Ther 1992; 261: 1260–7. PubMedCASGoogle Scholar
- Giembycz MA, Diamond J. Evaluation of kemptide, a synthetic serine-containing heptapeptide, as a phosphate acceptor for the estimation of cyclic AMP-dependent protein kinase activity in respiratory tissues. Biochem Pharmacol 1990; 39: 271–83. ArticlePubMedCASGoogle Scholar
- Torphy TJ, Rinard GA, Rietow MG, Mayer SE. Functional antagonism in canine tracheal smooth muscle: inhibition by methacholine of the mechanical and biochemical responses to isoproterenol. J Pharmacol Exp Ther 1983; 227: 694–9. PubMedCASGoogle Scholar
- Torphy TJ, Zheng C, Peterson SM, Fiscus RR, Rinard GA, Mayer SE. Inhibitory effect of methacholine on drug-induced relaxation, cyclic AMP accumulation, and cyclic AMPdependent protein kinase activation in canine tracheal smooth muscle. J. Pharmacol Exp Ther 1985; 233: 409–17. PubMedCASGoogle Scholar
- Sankary RM, Jones CA, Madison JM, Brown JK. Muscarinic cholinergic inhibition of cyclic AMP accumulation in airway smooth muscle. Am Rev Resp Dis 1988; 138: 145–50. ArticlePubMedCASGoogle Scholar
- Francis SH, Noblett BD, Todd BW, Wells JN, Corbin JD. Relaxation of vascular and tracheal smooth muscle by cyclic nucleotide analogs that preferentially activate purified cGMP-dependent protein kinase. Mol Pharmacol 1988; 34: 506–17. PubMedCASGoogle Scholar
- Heaslip RJ, Fiesa FR, Rimele TJ, Grimes D. Co-regulation of tracheal tone by cyclic AMP- and cyclic GMP-dependent mechanisms. J Pharmacol Exp Ther 1987; 243: 1018–26. PubMedCASGoogle Scholar
- Polson JB, Krzanowski JJ, Szentivianyi A. Inhibition of a high affinity cyclic AMP phosphodiesterase and relaxation of canine tracheal smooth muscle. Biochem Pharmacol 1982; 31: 3404–6. ArticleGoogle Scholar
- Bryson SE, Rodger IW. Effects of phosphodiesterase inhibitors on normal and chemically-skinned isolated airway smooth muscle. Br J Pharmacol 1987; 92: 673–81. ArticlePubMedCASGoogle Scholar
- Harris AL, Connell MJ, Ferguson EW, Wallace AM, Gordon RJ, Pagani ED, Silver PJ. Role of low Krm cyclic AMP phosphodiesterase inhibition in tracheal relaxation and bronchodilation in the guinea pig. J Pharmacol Exp Ther 1989; 251: 199–206. PubMedCASGoogle Scholar
- Shahid M, van Amsterdam RG, de Boer J, ten Berge RE, Nicholson CD, Zaagsma J. The presence of five cyclic nucleotide phosphodiesterase isoenzyme activities in bovine tracheal smooth muscle and the functional effects of selective inhibitors. Br J Pharmacol 1991; 104: 471–7. ArticlePubMedCASGoogle Scholar
- Small RC, Boyle JP, Duty S, Elliott KRF, Foster RW, Watt AJ. Analysis of the relaxant effects of AH 21–132 in guinea-pig isolated trachealis. Br J Pharmacol 1989; 97: 1165–73. ArticlePubMedCASGoogle Scholar
- Torphy TJ, Burman M, Huang LBF, Tucker SS. Inhibition of the low Km cyclic AMP phosphodiesterase in intact canine trachealis by SK&F 94836: mechanical and biochemical responses. J Pharmacol Exp Ther 1988; 246: 843–850. PubMedCASGoogle Scholar
- de Boer J, Philpott AJ, van Amsterdam GM, Shadid M, Zaagsma J, Nicholson CD. Human bronchial cyclic nucleotide phosphodiesterase isoenzymes: biochemical and pharmacological analysis using selective inhibitors. Br J Pharmacol 1992; 106: 1028–34. ArticlePubMedGoogle Scholar
- Qian Y, Naline E, Karlsson J-A, Raeburn D, Advenier C. Effects of rolipram and siguazodan on the human isolated bronchus and their interaction with isoprenaline and sodium nitroprusside. Br J Pharmacol 1993; 109: 774–8. ArticlePubMedCASGoogle Scholar
- Rabe KF, Tenor H, Dent G, Schudt C, Liebig S, Magnussen H. Phosphodiesterase isozymes modulating inherent tone in human airways: identification and characterization. Am J Physiol 1993; 264: L458–L64. PubMedCASGoogle Scholar
- Torphy TJ, Undem BJ, Cieslinski LB, Luttmann MA, Reeves ML, Hay DWP. Indentification, characterization and functional role of phosphodiesterase isozymes in human airway smooth muscle. J Pharmacol Exp Ther 1993; 265: 1213–23. PubMedCASGoogle Scholar
- Torphy TJ, Zhou H-L, Burman M, Huang LBF. Role of cyclic nucleotide phosphodiesterase isozymes in intact canine trachealis. Mol Pharmacol 1991; 39: 376–84. PubMedCASGoogle Scholar
- Small RC, Berry JL, Boyle JP, Chapman ID, Elliott KRF, Foster RW, Watt Ai. Biochemical and electrical aspects of the tracheal relaxant action of AH 21–132. Eur J Pharmacol 1991; 192: 417–26. ArticlePubMedCASGoogle Scholar
- Allen SL, Beech DJ, Foster RW, Morgan GP, Small RC. Electrophysiological and other aspects of the relaxant action of isoprenaline in guinea-pig isolated trachealis. Br J Pharmacol 1985; 86: 843–54. ArticlePubMedCASGoogle Scholar
- Fujiwara T, Sumimoto K, Itoh T, Suzuki H, Kuriyama H. Relaxing actions of procaterol, a ß-adrenoceptor stimulant, on smooth muscle cells of the dog trachea. Br J Pharmacol 1988; 93: 199–209. ArticlePubMedCASGoogle Scholar
- Honda K, Satake T, Takagi K, Tomita T. Effects of relaxants on electrical and mechanical activities in the guinea pig tracheal muscle. Br J Pharmacol 1986; 87: 665–71. ArticlePubMedCASGoogle Scholar
- Boyle JP, Tomasic M, Kotlikoff MI. Delayed rectifier potassium channels in canine and porcine airway smooth muscle cells. J Physiol 1992; 447: 329–50. PubMedCASGoogle Scholar
- Fleischmann BK, Washabau RJ, Kotlikoff MI. Delayed-rectifier potassium currents control resting membrane potential in ferret airway smooth muscle cells. J Physiol 1993; 469: 625–638. PubMedCASGoogle Scholar
- Kotlikoff MI. Potassium currents in canine airway smooth muscle cells. Am J Physiol 1990; 259: L384–L395. PubMedCASGoogle Scholar
- Kume H, Takai A, Tokuno H, Tomita T. Regulation of Ca 2+ -dependent-dependent K + -channel activity in tracheal myocytes by phosphorylation. Nature 1989; 341: 152–4. ArticlePubMedCASGoogle Scholar
- McCann JD, Welsh MJ. Calcium-activated potassium channels in canine airway smooth muscle. J Physiol 1986; 372: 113–27. PubMedCASGoogle Scholar
- Murray MA, Berry JL, Cook SJ, Foster RW, Green KA, Small RC. Guinea-pig isolated trachealis: the effects of charybdotoxin on mechanical activity, membrane potential changes and the activity of plasmalemmal K + -channels. Br J Pharmacol 1991; 103: 1814–18. ArticlePubMedCASGoogle Scholar
- Huang J-C, Garcia ML, Reuben JP, Kaczorowski GJ. Inhibition of ß-adrenoceptor agonist relaxation of airway smooth muscle by Ca 2+ -activated K + channel blockers. Eur J Pharmacol 1993; 235: 37–43. ArticlePubMedCASGoogle Scholar
- Jones TR, Charette L, Garcia ML, Kaczorowski GJ. Selective inhibition of relaxation of guinea-pig trachea by charybdotoxin, a potent Ca ++ -activated K + channel inhibitor. J Pharmacol Exp Ther 1990; 255: 697–706. PubMedCASGoogle Scholar
- Miura M, Belvisi MG, Stretton D, Yacoub MH, Barnes PJ. Role of potassium channels in bronchodilator response in human airways. Am Rev Respir Dis 1992; 146: 132–6. ArticlePubMedCASGoogle Scholar
- Carl, A, Kenyon JL, Uemura D, Fusetani N, Sanders KM. Regulation of Ca 2+ -activated-activated K + channels by protein kinase A and phosphatase inhibitors. Am J Physiol 1991; 261: C387–C92. PubMedCASGoogle Scholar
- Gunst SJ, Stropp JQ. Effect of Na-K adeninetriphosphatase activity on relaxation of canine tracheal smooth muscle. J Appl Physiol 1988; 64: 635–41. PubMedCASGoogle Scholar
- Takuwa Y, Takuwa N, Rasmussen H. Measurement of cytoplasmic free Ca 2+ concentration in bovine tracheal smooth muscle using aequorin. Am J Physiol 1987; 253: C817–27. PubMedCASGoogle Scholar
- Felbel J, Trockur B, Ecker T, Landgraf W, Hoffmann F. Regulation of cytosolic calcium by cAMP and cGMP in freshly isolated smooth muscle cells from bovine trachea. J Biol Chem 1988; 263: 16764–71. PubMedCASGoogle Scholar
- Itoh T, Izumi H, Kuriyama H. Mechanisms of relaxation induced by activation of ß-adrenoceptors in smooth muscle cells of the guinea pig mesenteric artery. J Physiol 1982; 326: 475–93. PubMedCASGoogle Scholar
- Mueller E, van Breeman. Role of intracellular Ca 2+ sequestration in β-adrenergic relaxation of smooth muscle. Nature 1979; 281: 682–3. ArticlePubMedCASGoogle Scholar
- Schied CR, Fay FS. β-adrenergic effects on transmembrane 45 Ca fluxes in isolated smooth muscle cells. Am J Physiol 1984; 246: C431–C8. Google Scholar
- Murray RK, Kotlikoff MI. Receptor-activated calcium influx in human airway smooth muscle cells. J Physiol 1991; 435: 123–44. PubMedCASGoogle Scholar
- Worley JF, Kotlikoff MI. Dihydropyridine-sensitive single calcium channels in airway smooth muscle cells. Am J Physiol 1990; 259: L468–L80. PubMedCASGoogle Scholar
- Murray RK, Fleischmann BK, Kotlikoff MI. Receptor-activated Ca influx in human airway smooth muscle: combined use of Ca imaging and perforated-patch clamp techniques. Am J Physiol 1992; 264: C485–C490. Google Scholar
- Hoth M, Penner R. Depletion of intracellular calcium stores activates a calcium current in mast cells. Nature 1992; 355: 353–6. ArticlePubMedCASGoogle Scholar
- Penner R, Matthews G, Neher E. Regulation of calcium influx by second messengers in rat mast cells. Nature 1988; 334: 499–504. ArticlePubMedCASGoogle Scholar
- Berridge MJ. Inositol trisphosphate and diacylglycerol: two interacting second messengers. Ann Rev Biochem 1987; 56: 159–93. ArticlePubMedCASGoogle Scholar
- Ehrlich BE, Watras J. Inositol 1,4,5-trisphosphate activates a channel from smooth muscle sarcoplasmic reticulum. Nature 1988; 336: 583–6. ArticlePubMedCASGoogle Scholar
- Ferris CD, Snyder SH. Inositol 1,4,5-trisphosphate-activated calcium channels. Ann Rev Physiol 1992; 54: 469–88. ArticleCASGoogle Scholar
- Danthuluri NR, Deth RC. Acute desensitization to angiotensin II. Evidence for a requirement of agonist induced diacylglycerol production during tonic contraction of rat aorta. Eur J Pharmacol 1986; 126: 135–39. ArticlePubMedCASGoogle Scholar
- Hall IP, Hill SJ. Beta-adrenoceptor stimulation inhibits histamine-stimulated inositol phospholipid hydrolysis in bovine tracheal smooth muscle. Br J Pharmacol 1988; 95: 1204–12. ArticlePubMedCASGoogle Scholar
- Hall IP, Donaldson J, Hill SJ. Inhibition of histamine-stimulated inositol phospholipid hydrolysis by agents which increase cyclic AMP levels in bovine tracheal smooth muscle. Br J Pharmacol 1989; 97: 603–13. ArticlePubMedCASGoogle Scholar
- Madison JM, Brown JK. Differential inhibitory effects of forskolin, isoproterenol, and dibutyryl cyclic adenosine monophosphate on phosphoinositide hydrolysis in canine tracheal smooth muscle. J Clin Invest 1988; 82: 1462–65. ArticlePubMedCASGoogle Scholar
- Hall IP, Donaldson J, Hill SJ. Modulation of fluoroaluminate-induced inositol phosphate formation by increases in tissue cyclic AMP content in bovine tracheal smooth muscle. Br J Pharmacol 1990; 100: 646–50. ArticlePubMedCASGoogle Scholar
- Offer GJ, Chilvers ER, Nahorski SR. β-Adrenoceptor induced inhibition of muscarinic receptor stimulated phosphoinositide metabolism is agonist specific in bovine tracheal smooth muscle. Eur J Pharmacol (Mol Pharmacol) 1991; 207: 243–48. ArticleCASGoogle Scholar
- Yang CM, Chou S-P, Sung T-C. Muscarinic receptor subtypes coupled to generation of different second messengers in isolated tracheal smooth muscle cells. Br J Pharmacol 1991; 104: 613–8. ArticlePubMedCASGoogle Scholar
- Conti MA, Adelstein RS. The relationship between calmodulin binding and phosphorylation of smooth muscle kinase by the catalytic subunit of 3′:5′ cAMP-dependent protein kinase. J Biol Chem 1981; 256: 3178–81. PubMedCASGoogle Scholar
- de Lanerolle P, Paul RJ. Myosin phosphorylation/dephosphorylation and regulation of airway smooth muscle contractility. Am J Physiol 1991; 261: L1–L14. PubMedGoogle Scholar
- Nishikawa M, de Lanerolle P, Lincoln TM, Adelstein RS. Phosphorylation of mammalian myosin light chain kinases by the catalytic subunit of cyclic AMP-dependent protein kinase and by cyclic GMP-dependent protein kinase. J Biol Chem 1984; 259: 8429–36. PubMedCASGoogle Scholar
- Nishikawa M, Shirakawa S, Adelstein RS. Phosphorylation of smooth muscle myosin light chain kinase by protein kinase C. J Biol Chem 1985; 260: 8978–83. PubMedCASGoogle Scholar
- de Lanerolle P, Nishikawa M, Yost A, Adelstein RS. Increased phosphorylation of myosin light chain kinase after an increase in cyclic AMP in intact smooth muscle. Science 1984; 223: 1415–1417. ArticlePubMedGoogle Scholar
- Obara K, De Lanerolle P. Isoproterenol attenuates myosin phosphorylation and contraction of tracheal muscle. J Appl Physiol 1989; 66: 2017–22. PubMedCASGoogle Scholar
- de Lanerolle P. cAMP, myosin dephosphorylation, and isometric relaxation of airway smooth muscle. J Appl Physiol 1988; 64: 705–9. PubMedGoogle Scholar
- Stull JT, Hsu LC, Tansey MG, Kamm KE. Myosin light chain kinase phosphorylation in tracheal smooth muscle. J Biol Chem 1990; 265: 16683–90. PubMedCASGoogle Scholar
- Miller JR, Silver PJ, Stull JT. The role of mysoin light chain kinase phosphorylation in beta-adrenergic relaxation of tracheal smooth muscle. Mol Pharmacol 1983; 24: 235–42. PubMedCASGoogle Scholar
- Tang D-C, Stull JT, Kubota Y, Kamm KE. Regulation of the Ca 2+ dependence of smooth muscle contraction. J Biol Chem 1992; 267: 11839–45. PubMedCASGoogle Scholar
- Clark RB, Friedman J, Dixon RFA, Strader CD. Identification of a specific site required for rapid heterologous desensitization of the beta-adrenergic receptor by cAMP-dependent protein kinase. Mol Pharmacol 1989; 36: 343–8. PubMedCASGoogle Scholar
- Hausdorff WP, Bouvier M, O’Dowd BF, Irons GP, Caron MG, Lefkowitz RJ. Phosphorylation sites on two domains of the beta 2-adrenergic receptor are involved in distinct pathways of receptor desensitization. J Biol Chem 1989; 264: 12657–12665. PubMedCASGoogle Scholar
- Benovic JL, Strasser RH, Caron MG, Lefkowitz RJ. Beta adrenergic receptor kinase: identification of a novel protein kinase that phosphorylates the agonist-occupied form of the receptor. Proc Natl Acad Sci 1986; 83:2797–801. ArticlePubMedCASGoogle Scholar
- Lohse MJ, Benovic JL, Caron MG, Lefkowitz RJ. Multiple pathways of rapid beta 2-adrenergic receptor desensitization. J Biol Chem 1990; 265: 3202–11. PubMedCASGoogle Scholar
- Kameyama K, Haga K, Haga T, Kontani K, Katada T, Fukada Y. Activation by G protein subunits of ß-adrenergic and muscarinic receptor kinase. J Biol Chem 1993; 268: 7753–58. PubMedCASGoogle Scholar
- Hall IP, Daykin K, Widdop S. ß-adrenoceptor desensitization in cultured human airway smooth muscle. Clin Sci 1993; 84: 151–7. PubMedCASGoogle Scholar
- Torphy TJ, Freese WB, Rinard GA, Brunton LL, Mayer SE. Cyclic nucleotide-dependent protein kinases in airway smooth muscle. J Biol Chem 1982; 257: 11609–16. PubMedCASGoogle Scholar
- Landgraf W, Hullin R, Gögel C, Hofmann F. Phosphorylation of cAMP-dependent protein kinase increases the affinity for cyclic AMP. Eur J Biochem 1986; 154: 113–7. ArticlePubMedCASGoogle Scholar
- Jiang H, Colbran JL, Francis SH, Corbin JD. Direct evidence for cross-activation of cAMP-dependent protein kinase by cAMP in pig coronary arteries. J Biol Chem 1992; 267: 1015–9. PubMedCASGoogle Scholar
- Lincoln, TM, Cornwell TL, Taylor AE. cGMP-dependent protein kinase mediates the reduction of Ca 2+ by cAMP in vascular smooth muscle cells. Am J Physiol 1990; 27: C399–C407. Google Scholar
- Hamaguchi M, Ishibashi T, Imai S. Involvement of charybdotoxin-sensitive K + channel in the relaxation of bovine tracheal smooth muscle by glyceryl trinitrate and sodium nitroprusside. J Pharmacol Exp Ther 1992; 262: 263–70. PubMedCASGoogle Scholar
- Robertson BE, Schubert R, Hescheler J, Nelson MT. cGMP-dependent protein kinase activates Ca-activated K channels in cerebral artery smooth muscle cells. Am J Physiol 1993; 265: C299–C303. PubMedCASGoogle Scholar
- Kume H, Graziano MP, Kotlikoff MI. Stimulatory and inhibitory regulation of calciumactivated potassium channels by guanine nucleotide-binding proteins. Proc Natl Acad Sci 1992; 89: 11051–5. ArticlePubMedCASGoogle Scholar
- Kume H, Kotlikoff MI. Muscarinic inhibition of singe KCa channels in smooth muscle cells by a pertussis-sensitive G protein. Am J Physiol 1991; 261: C1204–C9. PubMedCASGoogle Scholar
Author information
Authors and Affiliations
- Department of Inflammation and Respiratory Pharmacology, SmithKline Beecham Pharmaceuticals, King of Prussia, Pennsylvania, USA Theodore J. Torphy
- Division of Therapeutics, University Hospital, Queen’s Medical Centre, Nottingham, UK Ian P. Hall
- Theodore J. Torphy
You can also search for this author in PubMed Google Scholar
You can also search for this author in PubMed Google Scholar
Editor information
Editors and Affiliations
- Department Head Discovery Biology, Rhône-Poulenc Rorer Ltd, Dagenham Research Centre, RM10 7XS, Dagenham, Essex, England David Raeburn
- Department of Thoracic Medicine, Royal Brompton National Heart and Lung Institute, Dovehouse Street, SW3 6LY, London, England Mark A. Giembycz ( Lecturer ) ( Lecturer )
Rights and permissions
Copyright information
© 1994 Springer Basel AG
About this chapter
Cite this chapter
Torphy, T.J., Hall, I.P. (1994). Cyclic AMP and the Control of Airways Smooth Muscle Tone. In: Raeburn, D., Giembycz, M.A. (eds) Airways Smooth Muscle: Biochemical Control of Contraction and Relaxation. Respiratory Pharmacology and Pharmacotherapy. Birkhäuser, Basel. https://doi.org/10.1007/978-3-0348-7681-0_10
Download citation
- DOI : https://doi.org/10.1007/978-3-0348-7681-0_10
- Publisher Name : Birkhäuser, Basel
- Print ISBN : 978-3-0348-7683-4
- Online ISBN : 978-3-0348-7681-0
- eBook Packages : Springer Book Archive
Share this chapter
Anyone you share the following link with will be able to read this content:
Get shareable link
Sorry, a shareable link is not currently available for this article.
Copy to clipboard
Provided by the Springer Nature SharedIt content-sharing initiative